By Victor Lotocki, Ph.D. Student in the Seferos Research Group at the University of Toronto
It isn’t a surprise that the world has a plastic problem that impacts human and environmental health, yet we continue to over-rely on the material. In Canada, for example, plastic is found in 95% of manufactured goods, most of which are single-use.1 Recognizing the issue, in October 2020, Canada’s Minister of Environment and Climate Change announced the government’s plan to achieve zero plastic waste by 2030.2 Since only 9% of plastics are recycled in the country, Canada’s Zero Plastic Waste Agenda emphasizes ramping up recycling regulation and technology, and critically, many common single-use plastics including plastic checkout bags, straws, stir sticks, six-pack rings, and cutlery were also promised to be banned. Unfortunately, a recent report from Environmental Defense revealed that the Government of Canada will need to institute new substantial measures to increase the prevention, reuse, and recycling of plastics, as a million tonnes of waste will continue to be generated even in the best-case scenario.3 Due to current difficulties in managing its recycling as well as the dangers it poses to human and environmental health, polystyrene is a particularly relevant target for new recycling strategies and regulatory action.
Polystyrene is the most common waste item found in the South Atlantic, Indian, as well as Pacific oceans, where it accumulates in the Great Pacific Garbage Patch.4 More concerning is that of the major plastic pollutants, which also include polyethylene and polyethylene terephthalate, polystyrene is the fastest to degrade into microplastics,5 which have been linked to oxidative stress, inflammation, and metabolic disorders in humans.6 Styrene monomers and oligomers, which are anticipated carcinogens, in particular, have been found to leech out of polystyrene microplastics.7 This issue is also found closer to home. For example, in Lake Ontario, researchers have found an alarming 760 particles per kilogram of sediment sampled, with each of these particles being larger than 2 mm.8
Most polystyrene comes in the form of expanded polystyrene foam, which includes Styrofoam, and is notoriously difficult to recycle, with about 10% of it being recycled overall in Canada. What’s more, only 35% of communities within Canada even collect polystyrene, to begin with.9 Currently, the only province or territory in Canada in which every locality collects Styrofoam products is British Columbia, while most others vary by region.3 Manitoba and Prince Edward Island don’t collect polystyrene, while Nunavut has no plastic collection program in place at all. One of the challenges in collecting Styrofoam relates to its low density. Since most of its volume is air, it would take up a lot of space that could more efficiently be filled using denser plastics, and it could break apart in transit, contaminating other recyclables. As a result, many regions cannot justify the cost required for collection. Here in Toronto, we even have to pay companies such as to collect our polystyrene waste.9
Another issue with polystyrene, along with other common plastics, is in its starting material sourcing. The production of polystyrene is strongly tied to the petroleum industry, as the two key starting reagents, benzene, and ethylene, are generated from it through methods including steam cracking and catalytic reformation.10 Benzene is alkylated with ethylene in an acid-catalyzed reaction to form ethylbenzene, typically in the liquid phase with AlCl3 or zeolitic catalysts. Then, styrene is produced from ethylbenzene through catalytic dehydrogenation, before finally being polymerized into polystyrene. As over 99% of global ethylbenzene is used in this process, the production of the two is strongly linked.11 Overall, the manufacture of polystyrene is unsustainable from the very beginning of the process as it involves the emission of greenhouse gases and other pollutants (Figure 1). However, polystyrene production will be difficult to ramp down as it’s very cheap and will continue to be economical as long as crude oil and natural gas are in demand.
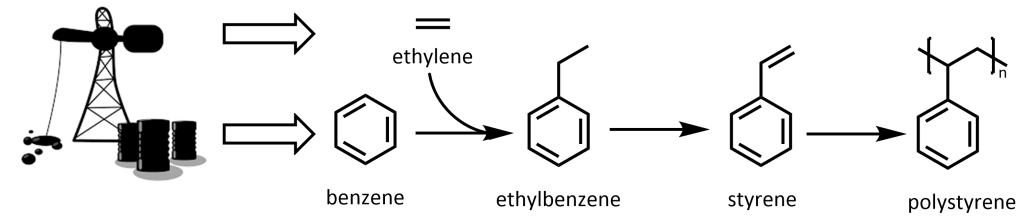
Figure 1. Synthesis of polystyrene from petroleum industry-sourced benzene and ethylene.
The other major issue associated with expanded polystyrene foam recycling stems from the fact that its lightweight cellular structure cannot be easily reproduced following the more common mechanical recycling methods; therefore, we need to turn to other methods for recycling. Pyrolysis, or thermal degradation, is the most widely studied form of degradation for plastics, and its advantages include being able to handle more contamination than mechanical recycling.12 Polystyrene pyrolysis is typically conducted at 350–700 °C, and more than 90% of the material by weight is consistently retained.10 Most of the material yielded by pyrolysis consists of styrene monomers and oligomers, allowing for reuse after repolymerization (Figure 2). The rate-determining step for the degradation of polystyrene through pyrolysis is β-scission on the polymer chain. However, this is mostly true for polystyrene containing many branching points, typically produced through radical polymerization. For higher-grade linear polystyrene synthesized using methods such as anionic polymerization or controlled radical polymerization, decomposition begins with scission at the chain end, generating the necessary radicals for further decomposition. Unfortunately, polymers with fewer branching points are also more thermally resistant, so they require higher temperatures, and therefore more energy-intensive conditions, for chains to begin breaking down.
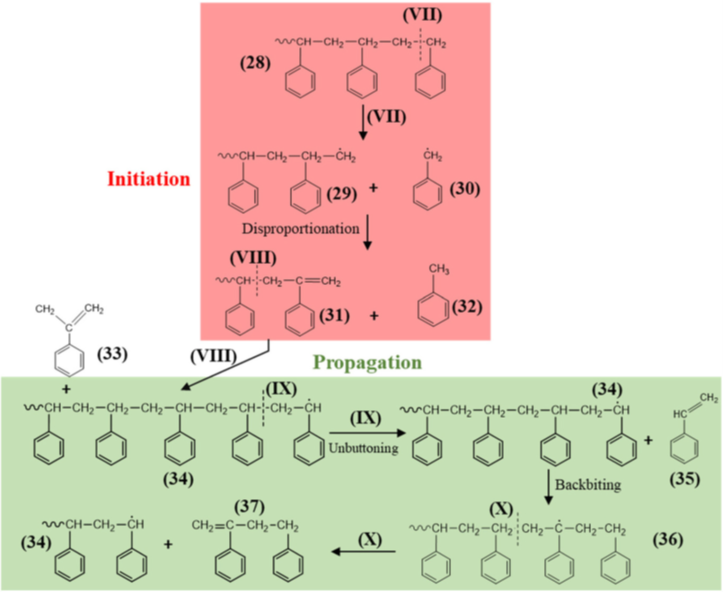
Figure 2. Thermal degradation of polystyrene. Reproduced from reference [10].
Although public facilities may be lagging behind, a few Canadian start-ups have fortunately stepped forward to try and tackle the polystyrene recycling problem. GreenMantra is a Brantford, Ontario-based start-up which has patented a method for the catalytic depolymerization of polystyrene using pyrolysis. Their recovered styrene monomers and other styrenic by-products have found commercial applications after repolymerization.13 In 2019, GreenMantra reached a joint development agreement with INEOS Styrolution and has begun providing their degraded polystyrene to replace a portion of INEOS Styrolution’s feedstock in manufacturing plastic goods, thereby creating a closed polystyrene cycle.14 More recently, INEOS Styrolution has also collaborated with Agilyx, based in Oregon, to open up a polystyrene recycling plant in Illinois that will be capable of recycling polystyrene contaminated with food back into food-grade plastics.15 In Montreal, Pyrowave is a company that uses a similar catalytic decomposition process but heated with microwave reactors that effectively decontaminate polystyrene from food and other organics, in forming styrene monomers for further use.16
Polystyvert is another Montreal-based company that has developed a rather different, yet arguably more effective way of recycling polystyrene. Instead of degrading it, Polystyvert uses cymene, an essential oil, which dissolves polystyrene, but not other contaminants that can then be filtered out.17 After separating the polystyrene from cymene using a patent-pending technology, the cymene can itself be recycled for later dissolution. Since dissolution only affects the state and not the chemical structure of polystyrene, the recycled material has virtually identical properties to the original product, and it can be re-foamed with a blowing agent. Crucially, this technology could address one of the largest current issues with expanded polystyrene foam recycling – the size and low density of the material. Immersing the material at the point of collection, or more realistically at the sorting facility, could give the economical impetus for polystyrene waste management needs.
All in all, green chemists are making decent progress in tackling the issues of polystyrene recycling. What’s left is for Canadian regulation to catch up. Right now, only British Columbia, Ontario, and Quebec have plans for systems that can reliably measure the amount of plastic waste that is collected, sorted, and sent to processing facilities, yet only Quebec has set targets for the amount of recycled material that should be used in new products.3 More importantly, the industrial sector, which is responsible for most plastic packaging waste, is not currently subject to legislation making it responsible for its own plastic products.
References
(1) The Role of Chemistry in a Circular Economy; Chemistry Industry Association of Canada, 2020. https://canadianchemistry.ca/wp-content/uploads/2020/07/The-Role-of-Chemistry_ENG_Web-FINAL.pdf.
(2) Canada One-Step Closer to Zero Plastic Waste by 2030; Environment and Climate Change Canada, 2020. https://www.canada.ca/en/environment-climate-change/news/2020/10/canada-one-step-closer-to-zero-plastic-waste-by-2030.html.
(3) Canada’s Zero Plastics Packaging Waste Report Card; Environmental Defence, 2022. https://environmentaldefence.ca/wp-content/uploads/2022/10/Environmental-Defence-Zero-Plastics-Waste-Report-Card_September-9-2022-October-7-2022.pdf.
(4) Eriksen, M.; Lebreton, L. C. M.; Carson, H. S.; Thiel, M.; Moore, C. J.; Borerro, J. C.; Galgani, F.; Ryan, P. G.; Reisser, J. Plastic Pollution in the World’s Oceans: More than 5 Trillion Plastic Pieces Weighing over 250,000 Tons Afloat at Sea. PLOS ONE 2014, 9 (12), e111913. https://doi.org/10.1371/journal.pone.0111913.
(5) Biber, N. F. A.; Foggo, A.; Thompson, R. C. Characterising the Deterioration of Different Plastics in Air and Seawater. Mar. Pollut. Bull. 2019, 141, 595–602. https://doi.org/10.1016/j.marpolbul.2019.02.068.
(6) Yee, M. S.-L.; Hii, L.-W.; Looi, C. K.; Lim, W.-M.; Wong, S.-F.; Kok, Y.-Y.; Tan, B.-K.; Wong, C.-Y.; Leong, C.-O. Impact of Microplastics and Nanoplastics on Human Health. Nanomaterials 2021, 11 (2), 496.
(7) Kwon, B. G.; Koizumi, K.; Chung, S.-Y.; Kodera, Y.; Kim, J.-O.; Saido, K. Global Styrene Oligomers Monitoring as New Chemical Contamination from Polystyrene Plastic Marine Pollution. J. Hazard. Mater. 2015, 300, 359–367. https://doi.org/10.1016/j.jhazmat.2015.07.039.
(8) Ballent, A.; Corcoran, P. L.; Madden, O.; Helm, P. A.; Longstaffe, F. J. Sources and Sinks of Microplastics in Canadian Lake Ontario Nearshore, Tributary and Beach Sediments. Mar. Pollut. Bull. 2016, 110 (1), 383–395. https://doi.org/10.1016/j.marpolbul.2016.06.037.
(9) Chung, Emily. Most Styrofoam Isn’t Recycled. Here’s How 3 Startups Aim to Fix That. CBC News. 2019. https://www.cbc.ca/news/science/styrofoam-chemical-recycling-polystyrene-1.5067879.
(10) Li, H.; Aguirre-Villegas, H. A.; Allen, R. D.; Bai, X.; Benson, C. H.; Beckham, G. T.; Bradshaw, S. L.; Brown, J. L.; Brown, R. C.; Cecon, V. S.; Curley, J. B.; Curtzwiler, G. W.; Dong, S.; Gaddameedi, S.; García, J. E.; Hermans, I.; Kim, M. S.; Ma, J.; Mark, L. O.; Mavrikakis, M.; Olafasakin, O. O.; Osswald, T. A.; Papanikolaou, K. G.; Radhakrishnan, H.; Sanchez Castillo, M. A.; Sánchez-Rivera, K. L.; Tumu, K. N.; Van Lehn, R. C.; Vorst, K. L.; Wright, M. M.; Wu, J.; Zavala, V. M.; Zhou, P.; Huber, G. W. Expanding Plastics Recycling Technologies: Chemical Aspects, Technology Status and Challenges. Green Chem. 2022, 24 (23), 8899–9002. https://doi.org/10.1039/D2GC02588D.
(11) Ullmann ́s Encyclopedia of Industrial Chemistry, 6th ed.; Wiley-VCH: 1999, 1999.
(12) Davidson, M. G.; Furlong, R. A.; McManus, M. C. Developments in the Life Cycle Assessment of Chemical Recycling of Plastic Waste–A Review. J. Clean. Prod. 2021, 293, 126163.
(13) Di Mondo, D.; Scott, B. Reactor for Treating Polystyrene Material. U.S. Patent 11,072,676.
(14) INEOS Styrolution and GreenMantra Sign JDA to Advance Polystyrene Chemical Recycling. INEOS Styrolution. Brantford, Ontario 2019. https://www.ineos-styrolution.com/news/ineos-styrolution-and-greenmantra-sign-jda-to-advance-polystyrene-chemical-recycling.
(15) INEOS Styrolution and Agilyx Advance Polystyrene Chemical Recycling Plant in Channahon, Illinois. INEOS Styrolution. Aurora, Illinois 2019. https://www.ineos-styrolution.com/news/ineos-styrolution-and-agilyx-advance-polystyrene-chemical-recycling-plant-in-channahon-illinois.
(16) Doucet, J.; Laviolette, J.-P. Catalytic Microwave Depolymerisation of Plastic for Production of Monomer and Waxes. U.S. Patent 11,518,864.
(17) Roland, C. Ô. T. É. Processes for Recycling Polystyrene Waste. U.S. Patent 11,407,878.